Valentime is an adult dating site that caters to couples who want to meet and mingle with people from other countries. It’s not just international relationships, either – it’ll let you hook up with local girls or guys from any country in the world! How...
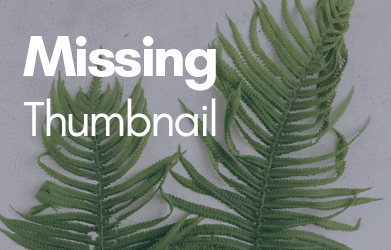